Why new eddy flux studies on the Colorado Plateau in Arizona?
Understanding and forecasting fluxes of carbon and water is a major goal of NEON's Integrative Research Program. Needed enhancements to the present network of flux tower sites were acknowledged in a recent review of the representativeness of the Ameriflux sites include "southern, southwestern, and Pacific Northwest environments" (Hargrove et al. 2003). Moreover, the same review noted that environments in the central, midwestern, and northeastern portions of the US are well represented currently. Thus, our proposed NEON Core Wildland Site at Beaver Creek in northern Arizona, as well as nearby sites which include tower-based instruments that have been measuring CO2 and H2O fluxes with the eddy covariance approach since late-summer 2005, would be a key enhancement to NEON.
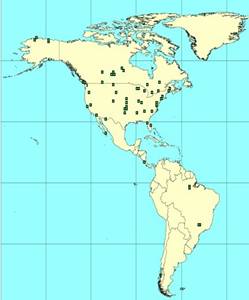
Figure 1 (left). The current distribution of tower-based sites using eddy covariance to measure fluxes of CO2 and H2O in the Ameriflux network (http://public.ornl.gov/ameriflux/). The green squares are current sites. There is a notable absence of sites on the Colorado Plateau in NEON Domain 13.
Sites of Opportunity for Understanding and Forecasting Impacts of Land Condition on Fluxes of Water, Energy, and Climate-Changing Gases
Current research by T. Kolb and colleagues (Northern Arizona University) provide "sites of opportunity" associated with the proposed Core site at Beaver Creek to investigate impacts of ecosystem disturbances and forest management activities on carbon and water fluxes and energy balance in forests dominated by ponderosa pine (Pinus ponderosa), the most widely distributed pine in western North America. The overall goal of this ongoing research is to understand controls on carbon, water, and energy fluxes by forest restoration treatments and wildfire in southwestern ponderosa pine forests. The specific objectives of the research are to: 1) compare ecosystem fluxes of carbon, water, and energy among control (untreated), restoration-treated, and wildfire-burned stands; 2) compare simulations of carbon and water fluxes from several biogeochemical models with empirical estimates from eddy covariance and biomass increment approaches; and 3) simulate effects of different climate change scenarios on carbon and water fluxes of southwestern ponderosa pine forests.
The current research by Kolb and colleagues includes three study sites near Flagstaff, Arizona that include the three, most-common stand conditions of southwestern ponderosa pine forests: unmanaged, managed, and burned by severe wildfire. They established tower-based micrometeorological equipment at all sites and have about 12 months of pretreatment data to support a novel, experimental comparison of impacts of a restoration thinning treatment that occurred in September, 2006, on fluxes of carbon, water, and energy, as well as impacts of a past, stand-replacing wildfire on fluxes. All sites occur at an elevation of about 2,200 m on soils derived from basalt (fine smectitic complex of frigid Typic Argiborolls and Mollic Eutroboralfs), the most common soil parent material in upland Southwestern forests. The Rocky Mountain variety of ponderosa pine (Pinus ponderosa var. scopulorum Engelm.) makes up over 90% of tree basal area currently in the unmanaged and restored stands, and this was true before burning killed all trees at the wildfire site.
Control, unmanaged stand: The stand (Fig. 2) is located on lands managed by the Northern Arizona University (NAU) Centennial Forest in cooperation with the Arizona State Lands Department. The stand occupies one section (267 ha) that has not been thinned nor prescribed burned for many decades; it is typical of unmanaged stands in ponderosa pine forests of the southwestern US. Most old-growth trees in the stand were harvested between 1914-16. The stand currently consists of trees from several regeneration cohorts; most overstory trees are about 87 years old. There are no immediate future plans for treatment of this site. Tree basal area in the stand averages about 30 m2 ha-1. Leaf area index is about 2.33 m2 m-2 (2.27 in overstory trees 0.06 in understory), which is close to the maximum estimated for southwestern ponderosa pine forests (McDowell et al. in press).
Figure 2 (left). Satellite photographs from early in year 2006, before the restoration thinning, for the control, restoration, and wildfire sites. All sites contain instrument towers for measuring fluxes of CO2, H2O, and energy; footprints shown are estimates based on prevailing wind and tower height. The thinning area at the restoration site was thinned in Sept. 2006, thus the thinning is not shown in the photograph.
Restoration stand: The stand (Fig. 2) is also located on the NAU Centennial Forest approximately 9 km from the unmanaged stand. Most of the overstory was cut between 1914-17. Heavy regeneration occurred several times starting in 1919 after the initial harvests, thus most trees in the stand are about 87 years old. The stand was lightly thinned by sales of sawtimber and pulp in 1951, 1956, 1965, 1987, and 1991. The NAU Centennial Forest managed the latest thinning in September 2006 which was designed followed restoration guidelines provided by the NAU Ecological Restoration Institute (Covington et al. 1997); these guidelines are commonly used for restoration and fuel reduction projects in northern Arizona (http://www.gffp.org/). The 2006 thinning occurred on 167 ha in the footprint of the instrument tower and focused on removal of thickets of small diameter trees; no trees with a diameter at breast height over 40 cm were cut.
Slash produced by the thinning was piled on site and will be prescribed burned in 2007 or 2008, depending on burning conditions. The thinning reduced tree density by 67% (465 to 154 trees ha-1), tree basal area by 39% (20.5 to 12.6 m2 ha-1), and leaf area index by 41% (1.6 to 0.9; all but 0.07 is overstory).
The restoration site is surrounded on all sides by the larger Woody Ridge Forest Restoration Project administered by the Coconino National Forest. The sections to the east of the study site were thinned to an average basal area of 11 m2 ha-1 in winter 2006 (Fig. 2), and the sections to the south and north are scheduled for thinning in 2007. Thus, the site is embedded in a larger, managed landscape to ensure similar land use and stand structure around the instrument tower.
Wildfire stand: This stand (Fig. 2) is located on the Coconino National Forest approximately 32 km from the unmanaged and restored stands and within the perimeter of the Horseshoe-Hochderffer fire complex that burned 10,500 ha of ponderosa pine forest in 1996. Prior to the fire the stand had a tree density of 343 ha-1, tree basal area of 31 m2 ha-1, and overstory leaf area index of 2.4, based on our measurements of adjacent unburned stands (n=10). The fire killed most trees within major portions of the burned area, and the site currently contains a community of herbaceous plants and a few shrubs. Natural regeneration of trees has not occurred in the tower footprint in the 10 years since the fire, which is common in southwestern ponderosa pine forest after severe fire (Savage and Mast 2005). Leaf area index averaged 0.62 in 2006 and is comprised entirely of grasses, other herbaceous plants, and a few shrubs. All trees in the tower footprint killed by the fire are present as standing snags or downed logs; salvage logging did not occur after the fire.
Role of Forest Disturbance in Fluxes of a Climate-forcing Gas, CO2
Evidence from pre-industrial periods (Brook 2005, Siegenthaler et al. 2005), industrial periods (Houghton et al. 2001, Osborn & Briffa 2006), and model simulations for the future (Houghton et al. 2001, Joos et al. 2001) all strongly suggest that temporal variation in temperature on Earth is linked to variations atmospheric concentrations of radiatively active ("greenhouse") gases, notably carbon dioxide (CO2). Recent analysis of temperature-sensitive proxy records shows that the middle- to late-20th century was the warmest period in the Northern Hemisphere in the last 1200 years and greenhouse gas concentrations were also highest over the same period (Osborn & Briffa 2006).
Terrestrial ecosystems strongly influence the global carbon (C) cycle and, combined with oceans, absorb about half of the CO2 currently released by human activities (Schimel et al. 2001, Dilling et al. 2003). Much of this C sink is estimated to occur in northern midlatitude regions (Potter & Klooster 1999), and is primarily due to photosynthesis exceeding respiration in terrestrial ecosystems. Simulated global patterns of C flux strongly suggest that forests dominated by ponderosa pine, including those represented by our study sites in northern Arizona, are a C sink (Potter & Klooster 1999). This sink influences regional and global climate and is of increasing interest to policy-makers concerned with management of greenhouse gases. Yet, it is widely acknowledged that estimates of the strength of the terrestrial C sink do not adequately consider impacts of ecosystem disturbance, such as wildfire or forest management activities, on CO2 fluxes (Breshears & Allen 2002, Hurtt et al. 2002, Schimel & Baker 2002, Thornton et al. 2002, Law et al. 2003, Litton et al. 2003, Dilling et al. 2003, Saleska et al. 2003, Law et al. 2004). Simulations and limited empirical data suggest that stand-scale CO2 and H2O fluxes depend strongly on stand age, disturbance, and forest age structure (Law et al. 2001, Thornton et al. 2002, Amiro et al. 2003, Law et al. 2003, Song & Woodcock 2003, Law et al. 2004, Pregitzer & Euskirchen 2004, Misson et al. 2005). Regional estimates of C sequestration by terrestrial ecosystems are likely overestimated because most studies avoid recently disturbed sites, and do not adequately consider impact of disturbance on C fluxes (Pacala et al. 2001, Breshears & Allen 2002, Hurtt et al. 2002, Saleska et al. 2003, Pregitzer & Euskirchen 2004). Elucidation of the magnitude, spatial and temporal scales, and biological processes of such influences is essential to improve estimates of exchanges of CO2, H2O, and energy between terrestrial ecosystems and the atmosphere in the western US.
Forest Disturbance, H2O, and Energy Balance
It is increasingly recognized that future climate of local regions will result from interactions between large-scale atmospheric circulation and fine-scale spatial variation in land cover and use characteristics (Diffenbaugh et al. 2005). Dramatic land-use changes, such as stand-replacing wildfire, can act as climate-forcing events by altering albedo and the partitioning of radiation between latent and sensible heat (Feddema et al. 2005, Jin & Roy 2005). However, the role of less dramatic land-use changes, such as modification of tree density and canopy cover by forest thinning activities, the use of which is widespread in the western US (Covington et al. 1997, Covington 2000, Lynch et al. 2000, Latham & Tappeiner 2002), on energy partitioning is scarcely considered in current climate change research. Understanding the role of land cover and use characteristics in energy balance of terrestrial ecosystems is needed given that increases in temperature driven by current rises in atmospheric CO2 concentration may feedback to reduce future terrestrial C uptake by decreasing gross photosynthesis in warm and dry regions, and by increasing respiration in cool regions (Joos et al. 2001, Gerber et al. 2004, Berthelot et al. 2005). Effects of direct radiant heating on land surface temperature are amplified by landscape conditions and climate factors that shift energy from latent heat to sensible heat (Diffenbaugh et al. 2005). Stand-replacing fires may produce such conditions, and these conditions may be countered by forest restoration practices that reduce the likelihood of stand-replacing wildfire and the impacts of drought on forest processes.
Figure 3 (left). Daily mid-day albedo (top), and partitioning of energy fluxes at our study sites where an intense, stand-replacing fire in 1996 replaced ponderosa pine forest with a grass- shrub-land (Fire, bottom) and a nearby, forested site (Control, middle). High albedo in winter is due to snow cover. Middle and bottom: SHF=soil heat flux, LE=latent heat flux, H=sensible heat flux, Net Rad=net radiation, Tot Solar Rad=total solar radiation.
Our preliminary results for 2005-2006 (Fig. 3, Montes-Helu et al. in preparation) on energy balance of a forested, unburned ponderosa pine forest (control) and a similar forest that was converted to a grass- shrub-land 10 years previously by a wildfire (fire) are consistent with the hypothesis that wildfire alters the partitioning of energy balance for at least a decade after burning. Total solar radiation was similar at both sites, yet net radiation was lower at the fire than the control site because of greater albedo at the fire site (Fig. 3, top). A greater proportion of net radiation was partitioned to sensible heat and the soil at the fire (Fig. 3 bottom) than the control site (Fig. 3 middle), but the magnitude of sensible heat was greater at the control site because of greater net radiation. The magnitude of heat flux to soil was greater at the fire than the control site. Overall, these preliminary results suggest warmer soil at the fire site, but greater warming of the local atmosphere at the control, forested site.
Partitioning of energy into latent and sensible heats is controlled by processes that influence the amount of H2O exposed to high vapor pressure deficit, and the conductance of H2O through soil and soil-plant-atmosphere pathways to locations with high vapor pressure deficit. Stand-level H2O flux in western coniferous forests is dominated by tree transpiration when trees have access to ample soil water and conductance from soil to leaf is high, but flux from understory plants and soil evaporation can increase in importance to at least 50% of total evapotranspiration (ET) when soil water availability and tree conductance are reduced during drought (Kurpius et al. 2003, Simonin et al. in press). Our recent work on the water balance of ponderosa pine forests in northern Arizona (Simonin et al. in press) show that drought and forest management thinning interact to influence components of ET, and likely the partitioning of energy between latent and sensible heats. During drought, thinning increased stand ET because overstory transpiration was limited by tree stomatal closure, and higher understory ET in the thinned stand was the dominant component of total-stand ET. During non-drought, thinning decreased stand ET because higher overstory transpiration in the unthinned stand with greater leaf area index was the most important component of ET. From these results we hypothesize that drought will drive the direction and magnitude of effects of restoration thinning on water and energy balances. During drought, the amount of radiation converted to sensible heat will be higher in unthinned than thinned forests because of greater stomatal limitation of latent heat flux in unthinned forests. The opposite pattern will occur in non-drought periods - the amount of radiation as sensible heat will be higher in thinned than unthinned forests - because of less capacity (leaf area) for latent heat flux in thinned forests. Thus, we predict that sensible heat and the land temperature of southwestern ponderosa pine forests will be driven by interactions between drought and forest management.
Forest Restoration
Contemporary concepts of forest restoration in interior forests of the western US center on producing stands with a low density of large-diameter trees, high abundance and diversity of native species, and a more natural fire-disturbance regime characterized by frequent, low-intensity fire (Covington et al. 1997, Lynch et al. 2000). Federal and local officials increasingly advocate forest restoration treatments because of improved forest condition, and reduced fuel loads and wildfire risk (Covington 2000, Malakoff 2002). The primary treatments used in restoration efforts are thinning of small diameter trees, followed by repeated controlled burns in the understory. These restoration treatments are typically applied to mature stands and produce stand structures that are very different from the younger ponderosa pine plantations that have been the subject of investigations of thinning effects on CO2 fluxes at the Blodgett Experimental Forest in California (Misson et al. 2005), and coniferous forests in the Pacific Northwest that are often intensively managed on short rotations (Law et al. 2004). Our understanding of the impacts of forest restoration treatments on ecosystem-level CO2 flux is limited. In two studies (Kaye & Hart 1998, Hart et al. 2006), restoration thinning generally stimulated soil respiration in association with higher soil temperature and greater production of grass litter, which decomposes faster than tree litter. A recent comparison between control, thinned, and composite (thinned + prescribed burned) treatments at the Pearson Natural Area near our study sites (Fig. 4 ) shows that net primary production (NPP) did not differ among treatments two and three years after treatment. NPP did not change because lower C fluxes to tree foliage and fine roots in thinned treatments were approximately balanced by higher fluxes to wood and herbaceous plants. However, we hypothesize that restoration treatments substantially reduce net ecosystem production (NEP), measured as NPP - heterotrophic respiration (RH), because decomposition of debris and roots of harvested trees (e.g., Pregitzer and Euskirchen 2004), and an increase in the amount of fast-decomposing herbaceous litter and in the decomposition rate of pine litter in thinned stands (Hart et al. 2005), will increase RH.
Figure 4 (left). Above-ground (ANPP) and below-ground (BNPP) net primary production did not differ significantly among untreated control, thinning restoration, and thinning+prescribed burning (composite) restoration treatments for the second (1995) or third (1996) post-treatment years at the Pearson Natural Area in northern Arizona (Kaye et al. 2005). We hypothesize that results will be different for NEP because thinning will stimulate RH, and thus reduce NEP compared with control, unthinned stands.
Wildfire
Over a century of fire suppression and heavy livestock grazing have altered ponderosa pine forests in the Southwest US, causing the natural low-intensity surface fire regime to disappear in most areas (Cooper 1960, Swetnam & Baisan 1996, Fulé et al. 1997). Ecosystem structure has shifted from open savanna-like forests to dense stands of small trees with little understory (Covington et al. 1994, Swetnam & Baisan 1996, Fulé et al. 1997). This shift is an important component of the recent C sink of terrestrial ecosystems (Potter and Klooster 1999, Houghton et al. 2000, Pacala et al. 2001, Schimel et al. 2001, Hibbard et al. 2003), but the sink is not sustainable because increasing C storage in woody fuels leads to catastrophic, stand-replacing wildfires in arid and semi-arid forests (Covington et al. 2001, Breshears & Allen 2002). Currently, about 50% of all federal forest lands in the interior western US are at high risk of large, uncontrollable, stand-replacing wildfire (GAO 1998, Graham et al. 2004). Since 1984, the average number of fires that burned over 400 ha in this region has increased by over 200%, and the area of forest burned has increased more than 400% (GAO 1998). Wildfire has been identified as one of the four major threats to National Forests in the US (USDA 2003). Stand-replacing wildfire is inevitable over large areas of western forests given current high fuel loadings (Covington et al. 1994). The 2002 Rodeo-Chediski Fire, which burned about 190,000 ha of ponderosa pine forests in Arizona (Finney et al. 2005), is a recent example. Attempts to measure or predict spatial variation in C sinks for the western US will have large errors if impacts of severe fire are not considered.
Large stand-replacing wildfires have enormous impacts on fluxes of CO2, H2O, and energy, yet understanding of these impacts is poor (Breshears & Allen 2002, Hurtt et al. 2002, Law et al. 2003, Law et al. 2004). Fire causes a sudden conversion of C stored in vegetation and soil to CO2, which is then released to the atmosphere. Such short-term effects of fire can be estimated from information on fuel consumption and extent of burning (Auclair & Carter 1993, Conard & Ivanova 1997, Harden et al. 2000, Page et al. 2002, Law et al. 2004). Longer-term effects of fire on C balance are more uncertain, and are influenced by fire effects on local hydrology, surface energy exchange, soil temperature (Amiro et al. 1999), rate of vegetation recovery (Amiro et al. 2003, Law et al. 2003, Litton et al. 2003), soil respiration, and erosion of soil organic carbon (Black & Harden 1994). Several studies show that NEP decreases in young, regrowing stands because decomposition of dead biomass produced from the disturbance results in RH exceeding NPP (Law et al. 2001, Law et al. 2004). Estimates of the number of years after stand-replacing disturbance required for a regrowing forest to shift from a source to a sink range from 2 to 5 years for pines in the southeastern US (Thornton et al. 2002), 10-30 years for ponderosa pine in the Pacific Northwest (Cohen et al. 1996, Law et al. 2001), and many decades for boreal forests (Schulze et al. 2000). Recovery of trees following stand-replacing wildfire appears to be much slower and more variable in southwestern ponderosa pine forests than other forested regions because of climatic differences (Savage and Mast 2005). Thus, measured and modeled dynamics of post-fire CO2 fluxes from more mesic forests or other regions (Thornton et al 2002, Amiro et al. 2003, Law et al. 2003, Litton et al. 2003, Kashian et al. 2006) may have limited application to southwestern ponderosa pine forests. Our study will provide unique data for the southwestern US by measuring fluxes of CO2, H2O, and energy during forest recovery from a stand-replacing wildfire in 1996 and modeling these fluxes as affected by fire and management treatments over several decades.
Trajectories of CO2 Exchange in Southwestern Ponderosa Pine Forests
Current interior ponderosa pine forests generally occur in one of three conditions that result in different trajectories of temporal variation in CO2 exchange. All conditions have a common history of increasing C sink strength and storage caused by suppression by European settlers of the frequent surface fires that used to characterize these forests. The first condition has been reported for a variety of forest ecosystems (Turner et al. 1995, Pregitzer and Euskirchen 2004, Kashian et al. 2006) and occurs in the absence of wildfire and forest management. Net ecosystem production increases with increasing leaf area and then declines (Fig. 5) due to a variety of mechanisms that reduce gross primary production or increase respiration (Ryan et al. 1997). This condition is common in current, unmanaged, unburned ponderosa pine forests, but increasing number, intensity, and size of intense stand-replacing wildfires in the last several decades (Westerling et al. 2006) show that it is not sustainable. The second condition is caused by stand-replacing fire that converts the forest to an early successional community. Carbon is lost rapidly to the atmosphere by combustion, and the land is converted from a C sink to a source (Fig. 5). The magnitude and duration of the C source depend on the density and rate of post-fire vegetation establishment (Kashian et al. 2006). Such stand-replacing fires in forests dominated by ponderosa pine often cause a type conversion from dense forest to either a grass/shrub community if trees do not rapidly establish, or a sparse forest if they do (Savage and Mast 2005). This conversion occurs because ponderosa pine does not have serotinous cones, and seedling establishment is slow in large burned areas due to the frequent asynchrony between good cone crop years and favorable conditions of seedling establishment (Savage et al 1996). Rapid post-fire replacement of a dense forest by another dense forest, as often occurs in forests that include trees with serotinous cones (Kashian et al. 2006), is uncommon in southwestern ponderosa pine forests. The vegetation-type conversion that occurs in the second condition has been widely recognized as a large, but poorly understood, influence on C uptake and storage (Harmon 2001, Kashian et al. 2006). The third condition is a managed forest where trees are thinned to reduce fuels and risk of stand-replacing fire. The thinning immediately reduces ecosystem leaf area and NEP (Fig. 5). However, the magnitude and duration of this reduction in NEP are largely unknown. The recovery of NEP could be rapid (Fig. 5 ) if growth of the residual trees and herbaceous plants increases rapidly after thinning (e.g., Kaye et al. 2005, Skov et al. 2005, Hart et al. 2006, Moore et al. 2006). However, recovery could be slow if CO2 release from heterotrophic decomposition of detritus produced by thinning, or on-site before thinning, balances or exceeds CO2 uptake by vegetation. Moreover, re-establishment of frequent fire after restoration thinning might result in fluctuations of CO2 release and uptake similar to the frequent fires that characterized ponderosa pine forests before disruption of the natural fire regime by Euro-American settlers (Fig. 5 ). Our proposed research will provide insight into these hypothesized trajectories of NEP by examining the direction and magnitude of change in NEP caused by stand-replacing wildfire and restoration thinning, as well as the key processes that drive such change.
Figure 5 (left). Trajectories of NEP for ponderosa pine stands where the frequent-fire regime was interrupted by fire suppression leading to an increase in NEP, followed by three possible scenarios: a) NEP peaks and gradually declines in the absence of management and wildfire; b) Intense wildfire rapidly converts the C sink to a source to the atmosphere, followed by unknown rates of recovery; c) Restoration thinning is used to avoid stand-replacing wildfire by reducing fuels, followed by unknown effects on NEP and rates and trajectories of recovery.